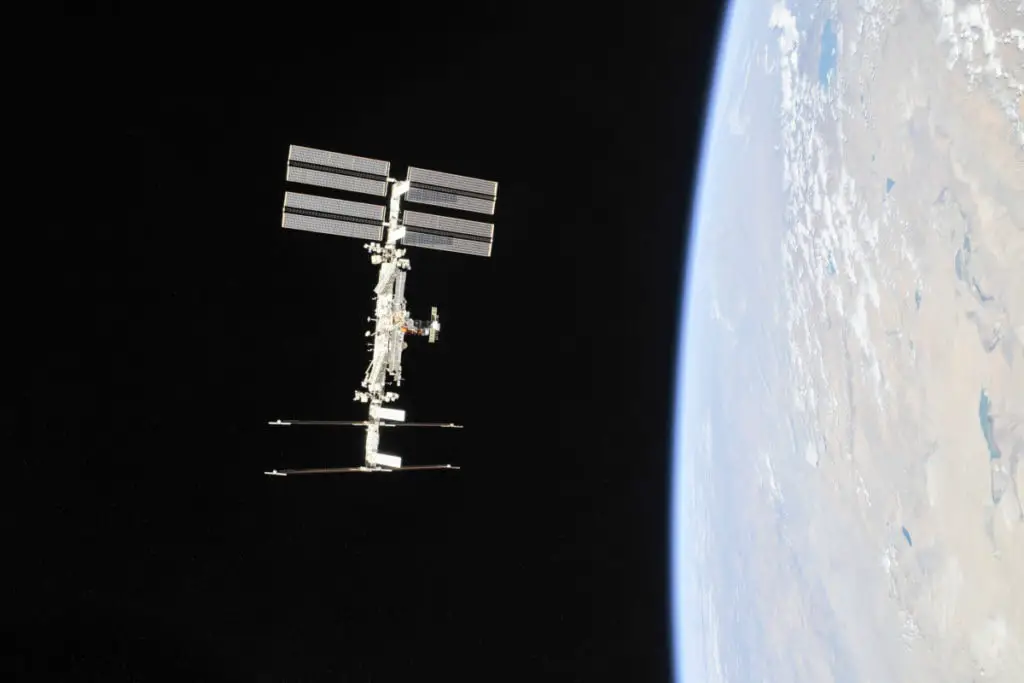
Intriguingly, much of the population on Earth acknowledges the International Space Station’s existence, but not many actually understand how it works. With the possibility of a commercial space station retiring the ISS in the near future, our responsibility is to learn about and pay due respect to the spacecraft that has fueled our dreams and ambitions to explore the unknown.
The International Space Station is made of multiple modules launched and assembled in space within a 22-year period. Each of the components contributes to the habitability of astronauts and different areas of scientific exploration under microgravity. This includes logistics, power, life support components (water supply, oxygen), research modules, cargo carriers, and robotic arms.
Read on to find out how all these components fit together to create the International Space Station we know today.
What is the International Space Station?
The International Space Station, otherwise known as the ISS, is the largest spacecraft in orbit around Earth. Russia launched the first piece of the ISS in 1998, and it serves as a unique research facility with state-of-the-art laboratories for conducting experiments under microgravity conditions.
ISS modules were often launched from different countries and continents, and they were not assembled until reaching orbit.
By orbiting the Earth in its low Earth orbit (LEO) at an approximate altitude of 250 miles traveling at a speed of 5 miles per second, or 17,500 miles per hour, the ISS can make a trip (orbit) around the Earth every 90 minutes.
This allows the ISS to complete 16 orbits around the Earth every 24 hours while traveling through 16 sunrises and sunsets.
The ISS results from an international partnership of space agencies. The principal space agencies include the United States, Russia, Europe, Japan, and Canada.
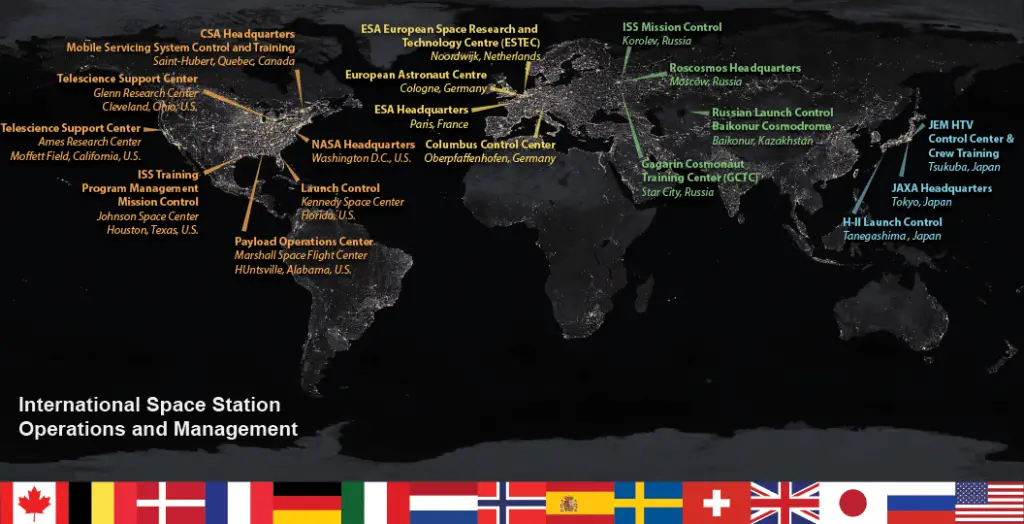
The following is a list of all international partners involved with the International Space Station:
- United States (NASA)
- Russia (Roscosmos)
- Canada (CSA)
- Japan (JAXA)
- Europe (ESA)
- Belgium
- Denmark (DNSC)
- France (CNES)
- Germany (DLR)
- Italy (ASI)
- Netherlands
- Norway (NSC)
- Spain (INTA)
- Sweden (SNSB)
- Switzerland
- United Kingdom
What is the purpose of the International Space Station?
The international space station is a technological marvel that is also the most ambitious international collaboration project and the most politically complex space exploration program to exist.
The experiments conducted aboard the ISS can help humans understand how to best adapt to long-term space living and develop the technology needed to help us explore further and further into the unknown.
These technologies and the study of biological and physical processes in the near absence of gravity enable the creation of products called “spinoffs“, which are used to benefit the people on Earth.
Examples of “spinoffs” include the support of water purification efforts worldwide, where the technology developed for water purification in space can be used for high-risk areas on Earth to provide safe drinking water. Robotic arms for maneuvers in space also make the impossible possible by removing inoperable brain tumors that could not have been accomplished with human hands alone.
As astronauts were losing 1.5% of their total bone mass density per month, we were able to identify mechanisms that control bones at a cellular level, as well as the diet and exercises best used for bone loss prevention, which can be applied to vulnerable populations on Earth that suffer from diseases like osteoporosis.
The existence of the ISS also paved the way for the commercialization of LEO by allowing commercial research and payloads to come onboard. NASA’s willingness to contract commercial cargo resupply and crew transportation services give U.S.-based companies a competitive edge. This, in turn, not only boosts the U.S. space economy but also frees up NASA’s funding to focus on missions involving deep space exploration.
How big is the International Space Station?
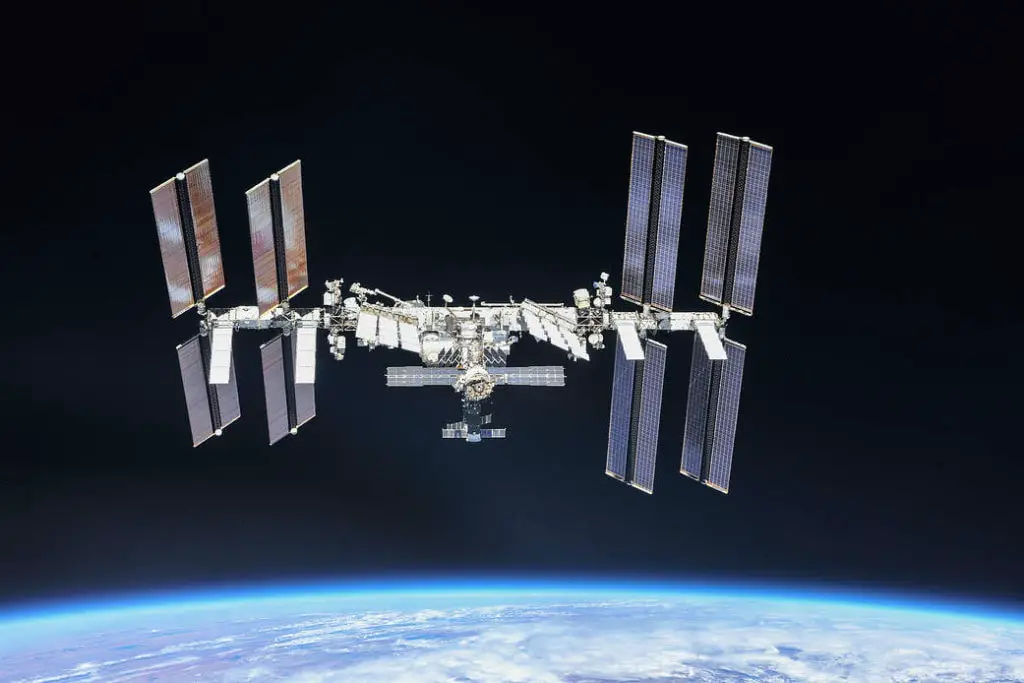
The ISS measures 357 feet from end to end, the distance one yard shy of a full-length American Football field that includes its endzones! Its solar arrays span 240 feet wide, which is around the same length as the world’s largest passenger aircraft, the Airbus A380.
Weighing at approximately 400 tonnes, or more accurately 419,725 kilograms (925,335 pounds), the ISS hides eight miles of wire connecting its electrical systems.
This gigantic spacecraft contains living and working space larger than a six-bedroom house, including six sleep quarters, two bathrooms, one gym, and a 360-degree view bay window.
Modules of the International Space Station
The assembly of the International Space Station is much like the construction of a Lego block set. With 39 main modules, the ISS was intricately assembled, mission by mission, over 22 years in LEO.
The modules can be compartmentalized into the following systems:
- The Integrated Truss Structure consists of 12 trusses that form the backbone of the ISS. There are attachment points for components such as solar panels, thermal control radiators, and external payloads. The ITS also provides mobile transporter rails to enable work outside the space station.
- Nodes are connecting modules between different segments of the ISS. They also provide life support systems, storage space, crew quarters, and exercise machines!
- Pressurized Modules of the ISS include crew quarters, laboratories, servicing modules, etc., and provide a habitable environment for the astronauts that call the space station home.
- The ISS’s Internal Research Accommodations include six labs that allow for research in human physiology, biology, physics, materials, fluid mechanics, etc., under microgravity conditions.
- The ISS’s External Research Accommodations include workstations mounted to the outside of the ISS for experiments that require the vacuum-like environment of space.
- The Mobile Servicing System consists of three modules: the Mobile Base System, Canadarm2, and Dextre. The service platform along the truss rails and robotic arms are often used for maneuvering modules, spacecrafts, and astronauts around the station.
- Airlocks are areas where payloads can be transferred into the station, as well as depressurized environments where astronauts and cosmonauts can prepare and service their spacesuits for extravehicular activities such as spacewalks.
For a detailed overview of all modules aboard the ISS, check out the article “Every Module of the International Space Station Explained“.
How does the ISS get water?
Humans cannot survive in space without water. As such, without the Earth to provide, the ISS must obtain water through artificial means.
Each astronaut needs approximately a gallon of water per day for consumption, food preparation, and hygiene.
The ISS receives its water supply by utilizing the following methods:
The Environmental Control and Life Support System (ECLSS) Water Recovery System (WRS) consists of two subsystems: the Urine Processor Assembly (UPA) and Water Processor Assembly (WPA).
The WRS recycles wastewater like urine through the urine recovery system (the toilet), cabin humidity condensates through vents that suck in the sweat and carbon dioxide, as well as the waste inside the Extravehicular Activity Suit’s hydration system.
Water produced by the urine processor is sent to the water processor with all other wastewaters for treatment. Inside the WPA, a series of multi-filtration beds and a catalytic oxidizer is used for purification; the electrical conductivity sensors in the system then check the water for its purity.
Water that does not pass the purity check is reprocessed, while clean water is sent to a storage tank. The current Water Recovery System can recover and recycle about 90% of the water on the ISS.
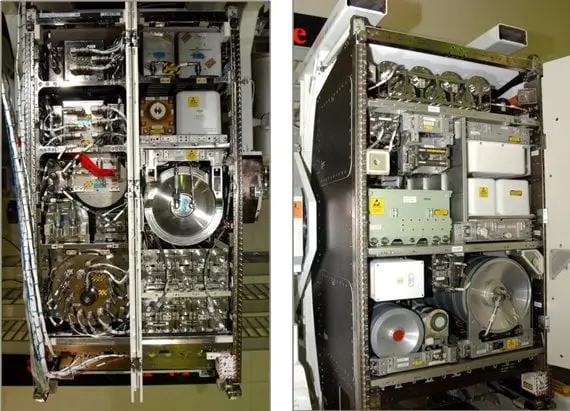
Image by NASA
The Sabatier System also plays an important role in minimizing the size and weight of life support systems aboard the ISS. This system uses a catalyst that reacts with carbon dioxide and hydrogen to produce water and methane.
This system is vital as carbon dioxide and hydrogen is the byproducts of astronauts and the life-support systems onboard the ISS. Both gases in excess were vented out into space before the system’s installation in 2010.
The Sabatier System has effectively closed the loop in the oxygen and water regeneration cycle by sharing a vent line with the Oxygen Generation System to obtain hydrogen, and provided one more way to produce water on the station without transporting it from Earth.
Iodine compatible water containers (ICWCS) are soft containers used for fluid collection and stowage applications on the ISS and other shuttles. Despite the systems available onboard the station to produce potable water, ionized water is still supplied to the ISS via:
- SpaceX’s Dragon
- Orbital’s Cygnus
- JAXA’s H-II Transfer Vehicle (HTV)
- Russian Progress
How does the ISS get air and oxygen?
Like water, this ingredient of life can only be obtained through artificial means in space. As such, breathable air is provided on the ISS through several systems:
The Environmental Control and Life Support System (ECLSS) Air Revitalization System (ARS) is dedicated to removing particulates, microorganisms, and volatile organic trace gases produced by electronics, plastics, and human off-gassing, including carbon dioxide exhaled by the crew.
Consisting of three separate units, the ARS flows the cabin air through an activated charcoal bed to remove high molecular weight organics and potential catalyst poisons. The air is then sent to a catalytic oxidizer to destroy low molecular weight compounds not captured by the charcoal bed. The lithium hydroxide bed finally removes any acid produced by the oxidation of hydrocarbons that might have broken through the charcoal bed.
A molecular sieve is used to remove carbon dioxide by separating and capturing different gases based on their size.
With the cabin air free from contaminants, the Environmental Control and Life Support System (ECLSS) Oxygen Generation System (OGS) produces the oxygen for the crew to breathe.
This system consists of two assemblies:
- Oxygen Generation Assembly (OGA)
- Carbon Dioxide Reduction Assembly (CDRA) of the Sabatier System
The oxygen generation assembly is made of cell stacks that electrolyze (break apart) the Water Recovery System’s water, which produces oxygen and hydrogen as byproducts. The oxygen is delivered to the cabin, while the hydrogen is either vented into space or fed to the carbon dioxide reduction assembly of the Sabatier system.
Like the Air Revitalization System, the carbon dioxide removal assembly also utilizes a molecular sieve in the form of a synthetic rock called zeolite, which causes the carbon dioxide and water to stick on while everything else passes through. The filtered CO2 is compressed and stored in tanks until enough hydrogen is available from the Oxygen Generation Assembly.
When the OGA is operational to produce hydrogen, with enough CO2 available, the Sabatier system activates to produce methane and water via the Sabatier reaction (CO2 + 4H2 <-> CH4 + 2H2O).
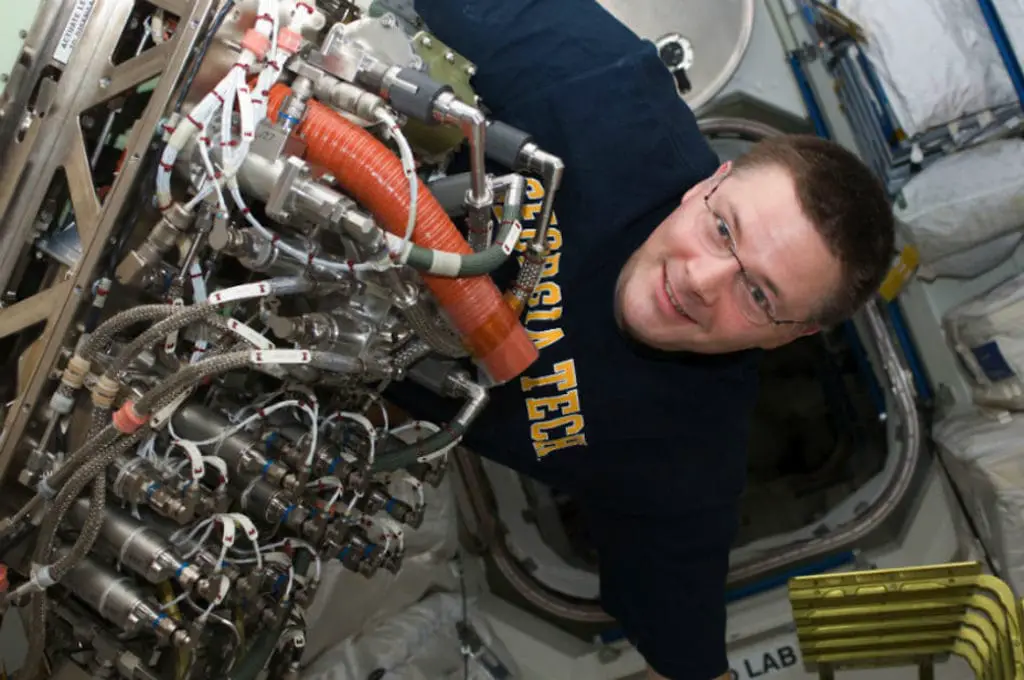
Image by NASA
Despite having the ability to produce breathable air, the ISS also requires Oxygen and Nitrogen stores to provide for atmosphere replenishment, payload operations, as well as supplying the gases needed to fill the station’s airlocks for spacewalks. The tanks for the Nitrogen/Oxygen Recharge System (NORS) are supplied by:
- SpaceX’s Dragon
- Orbital’s Cygnus
- JAXA’s H-II Transfer Vehicle (HTV)
- Russian Progress
The Solid Fuel Oxygen Generator aboard the ISS is a backup system that burns candles to provide oxygen.
How does the ISS stay warm?
It often goes without saying, the Sun does a very decent job of keeping us warm on Earth. But what about in space?
The temperature on the side of the ISS facing the Sun can reach up to 121 degrees Celsius, while the temperature of the side facing away from the Sun can drop to -157 degrees Celsius. With this almost 300 degrees Celsius difference, the ISS had to be designed to keep both the astronauts and equipment aboard at a comfortable and stable temperature environment.
The Thermal Control System (TCS) aboard the ISS was designed with this specific purpose in mind. The Passive Thermal Control System (PTCS) of the TCS consists of four components that maintain ISS temperature within defined limits:
- Insulation
- Surface Coating
- Heaters
- Heat Pipes
The insulation used on the ISS is the most common passive thermal control element used on spacecrafts on Multilayer Insulation (MLI). It consists of an outer layer, an interior layer, and an inner cover layer. The purpose of the MLI is to keep the solar radiation out of the ISS, and the cold from penetrating the station.
- The outer layer is generally made of opaque materials that generate a low amount of particulate contaminants, which can also survive the environments the ISS is exposed to. Common materials used are different types of synthetic polymers Teflon and Kapton to protect the interior layer.
- The interior layer needs to have low emittance, which is often made with aluminized Mylar to protect against solar radiation.
- The inner cover is the layer facing the ISS hardware which is also used to keep the Mylar sheets separated so heat does not conduct between layers. It is made with non aluminized material such as Dacron and Nomex to avoid short-circuiting the electrical system.
The surface coating used on the ISS are often materials that can change the thermo-optical properties of external surfaces.
The heaters of the ISS were actually not meant to keep the astronauts warm, but the hardware. These heaters are used with thermostats or solid-state controllers to keep a component at a specific temperature or warm-up components to their minimal operating temperatures.
Heat pipes onboard the ISS are a closed two-phase liquid-flow cycle that consists of a condenser and evaporator. This system can transport large quantities of heat without electrical power.
With how well the ISS is insulated, it actually poses another challenge… IT GETS TOO WARM!
It is great that this massive orbiting laboratory is stocked with many heat-producing instruments and electrical hardware that keeps the station operational. Still, almost every watt of power would end up as heat, and something has to be done to get rid of the excess.
To counteract this problem, the ISS designers created the Active Thermal Control System (ATCS) that utilizes heat exchangers and cold plates, where the air and water heat exchangers cool and dehumidifies the internal atmosphere, and high heat generators are cooled on custom-built cold plates.
The water-based internal cooling loops run through the heat exchangers and cold plates to remove the generated heat loads. This heat is then transferred to radiators on the exterior of the station. However, to prevent the water circulating the pipes from freezing as it exits the station, the waste heat is exchanged a second time to a loop containing ammonia on the radiator, as ammonia freezes at a lower temperature (-77 degrees Celsius).
How does the ISS get electric power?
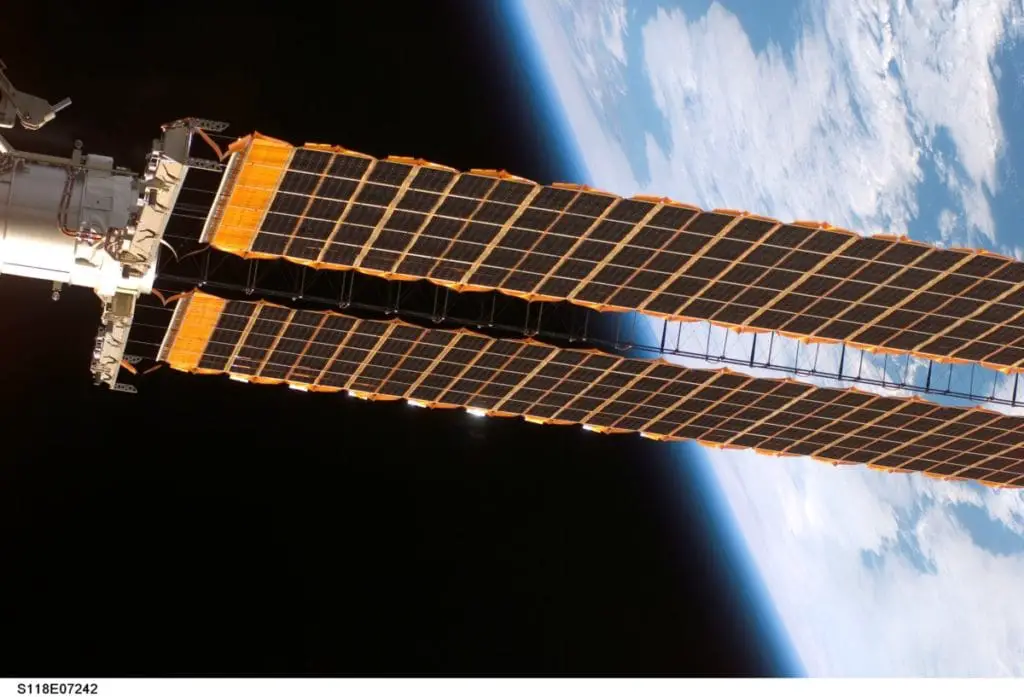
The Electrical Power System (EPS) aboard the ISS generates, stores, and distributes power and secondary power to its users. Its components include:
- Each Solar Array Wing (SAW) includes 2 blankets of 32,800 solar cells. They convert sunlight to DC power at 14% efficiency and produce a maximum of 31kW at the beginning of its life cycle and degrading to 26kW after 15 years.
- Beta Gimbals are used to track the seasonal changes of the Sun.
- Solar (Array) Alpha Rotation Joint (RARJ) tracks the Sun’s direction through the ISS’s trip across Earth’s orbit.
- Electronic Control Unit (ECU) controls the pointing of the SAW.
- Sequential Shunt Unit (SSU) maintains the constant voltage output at 160 Volts.
- DC-to-DC Converter Units (DDCUs) converts the primary 160 V power to a secondary 124 V power.
- Main Bus Switching Units (MBSUs) routes power to their intended locations in the ISS.
- Direct Current Switching Unit (DCSU) routes power from the solar arrays to the MBSUs.
- Nickel-Hydrogen/Lithium-ion Batteries stores electrical energy harvested by the SAWs. All Nickle-Hydrogen batteries have been swapped out for Lithium-ion batteries since 2017 due to their higher level of specific energy.
- Remote Power Controllers (RPCs) control the flow of electric power to users.
How does the ISS get food?
The astronauts aboard the International Space Station receives the bulk of their food through resupply missions that were planned years in advance. This mission manifest has to be followed as to ensure the crew aboard the ISS are always stocked and prepared.
The U.S. and Russia each provide their half of the food, while other space agencies such as ESA, JAXA, and CSA bring their own specialities that can be shared amongst all crew members.
The Space Food Systems Laboratory at the Johnson Space Centre in Houston is responsible for all the testing, preparation, and packaging of U.S. food to be delivered to the ISS.
This process starts with a regular day of grocery shopping at a supermarket, where the ingredients for the meals are bought in accordance with what the astronauts have ordered from a standard menu containing 250 options. These options can often be augmented with some personal choices or family deliverables.
Unfortunately, it is expensive to send cargo up into space, so most meals have to be dehydrated to remove 97% of their water content. There is also no dedicated freezer or refrigerator on the ISS other than a chiller, so all foods have to be stored and remain stable at ambient temperatures. As such, the lack of moisture will prevent spoilage. Each portion of the final product weighs approximately 1 ounce.
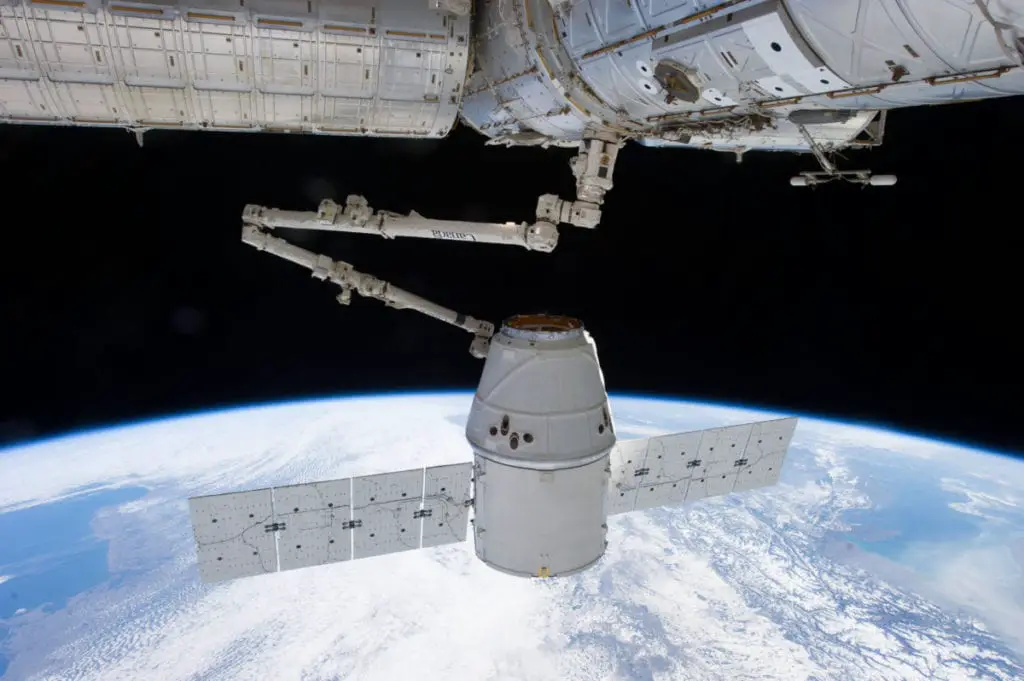
Image by NASA
All space food are shipped off to one of three launch sites for loading into resupply modules:
- Cape Canaveral Airforce Station (Florida) aboard SpaceX Dragons
- Mid-Atlantic Regional Spaceport (Georgia) aboard Cygnus Spacecraft
- Tanegashima Space Center (Japan) aboard HTVs
All modules carry approximately 3.8 pounds of food and 1 pound of packaging per astronaut for each mission day. (three meals a day with snacks)
A backup safe haven food system is also available to provide an extra 2000 calories per day per astronaut. This was designed to sustain the crew for an extra three weeks in case of emergencies
The following are a list of all types of space food available:
- Beverages are provided as freeze-dried drinks vacuum-sealed in beverage pouches. This might include coffee, tea, lemonade, orange juice, etc.
- Fresh Foods are foods like fresh fruits, vegetables, and tortillas delivered by resupply missions. Due to their short shelf life, they need to be eaten within the first two days of arrival to prevent spoilage.
- Irradiated Meat such as beef steaks is sterilized with ionizing radiation to prevent spoilage.
- Intermediate Moisture is foods like beef jerky that have some moisture but not enough to spoil immediately.
- Natural Form are commercially available shelf-life stable foods such as nuts, cookies, and granola bars.
- Rehydratable Foods have been dehydrated via heat, osmotic, or freeze-drying, which can be rehydrated with hot water before consumption.
- Thermostabilized foods have undergone the retort process that heats foods to destroy pathogens, microorganisms, and enzymes that may cause spoilage.
- Extended Shelf-Life Bread Products are foods like scones, waffles, and rolls specially formulated to have a shelf life of up to 18 months.
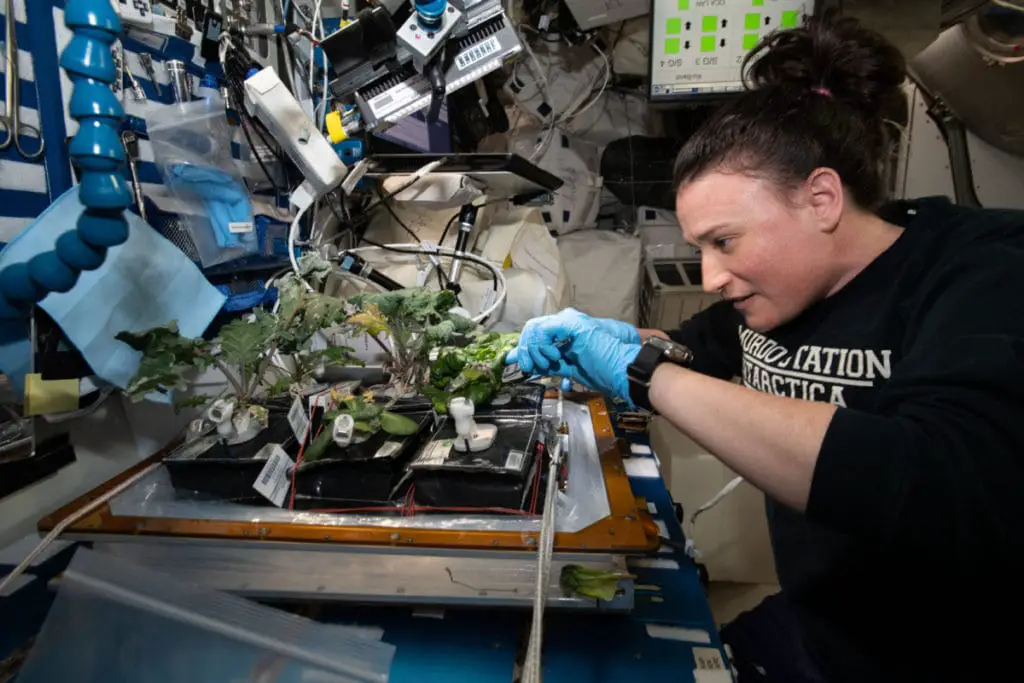
Image by ESA/Alexander Gerst
Many research experiments are currently underway aboard the ISS to examine the possibility of plant growth in space. However, only one of these systems has produced edible vegetables – The Vegetable Production System:
- Size of a carry-on piece of luggage that holds six plants.
- The plants are grown in a “pillow” filled with clay-based growth media and fertilizer.
- These pillows are used to distribute water, nutrients, and the air around the roots, otherwise due to microgravity conditions, the roots would be engulfed by air drowned in water as fluids tend to form bubbles.
- This system has successfully grown three types of lettuce: Chinese cabbage, mizuna mustard, red Russian kale and zinnia flowers.
- Some of the harvests were eaten by astronauts, and the rest were returned to Earth to be sampled.
- Helps NASA study plant growth in microgravity conditions.
- Adds fresh food to the astronaut’s diet to enhance their happiness and well-being.
How does the ISS communicate with Earth?
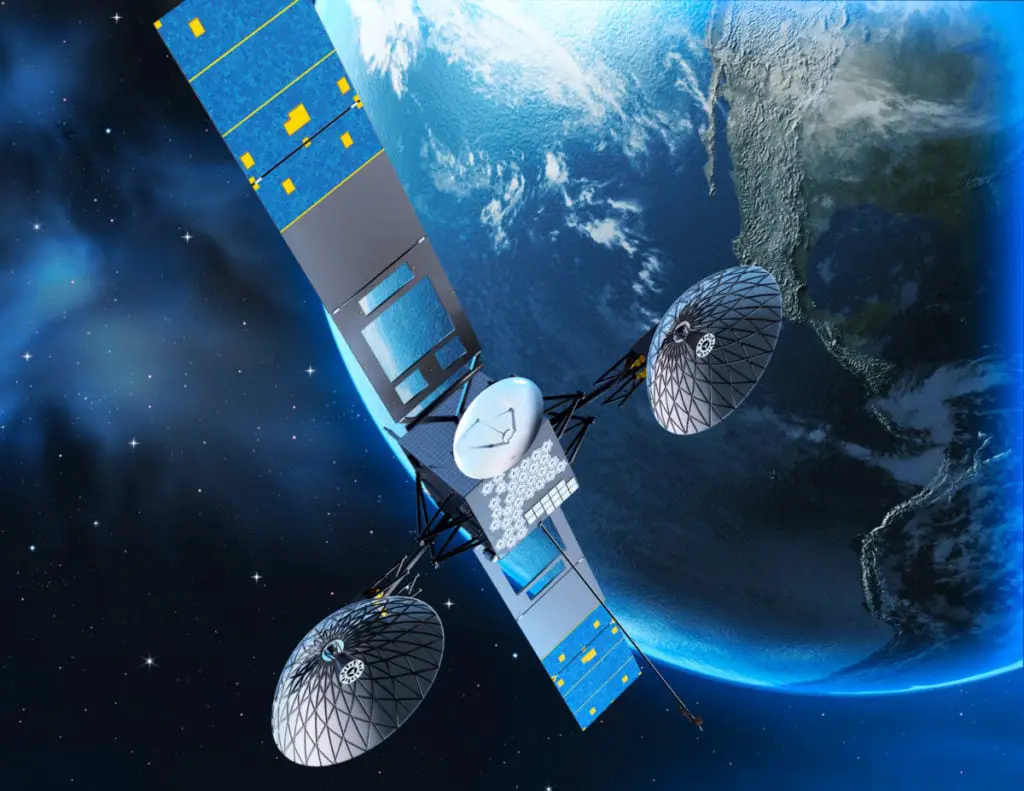
Image by NASA
The Communication & Tracking System (C&T) of the ISS utilizes six communication systems to remain in contact with Earth [1]:
- S-Band Subsystem transmits voice, files, commands, and telemetry between the ISS and the Mission Control Centre in Houston (MCC-H) via the Tracking & Data Relay Satellite System.
- Ku-Band Subsystem is used for downloading high rate data such as video and payload (experiment) data.
- Audio Subsystem distributes voice and caution/warning tones onboard the ISS
- Ultrahigh Frequency (UHF) Subsystem provides voice and data recognition between the ISS, the Orbiter, and the Extravehicular Activity (EVA) suited crewmembers.
- Video Distribution System (VDS) distributes video onboard the station through fiber optic-analog lines, and interfaces with the Ku-band Subsystem to send videos to the ground stations.
- Russian On-orbit segment (ROS) Communication System uses the Lira system for two-way communication between the ISS and Mission Control in Russia via the Luch relay satellite constellation.
How does the ISS move and stay in orbit?
The Guidance, Navigation, and Control System (GN&C) control the ISS’s altitude and orientation with respect to Earth and the Sun at all times. This is very important as the location of the ISS is imperative for maintaining thermal, power, microgravity levels, and communications.
Both the United States and Russia contribute to the system with:
- U.S. Segment
- GPS Receiver and Antennas provide information about the location of the ISS.
- Rate Gyro Sensors provide information about the change in orientation of the ISS.
- Control Moment Gyroscopes (CMG) is the main method of altitude control, which consists of a flywheel that spins at 6600 revolutions per minute. As the flywheel is repositioned through the use of a gimbal, its built-up angular momentum enables the ISS to move to new altitudes. The CMG is advantageous over thrusters as it relies only on electric power, which allows for smooth and continuously variable altitude control.
- Russian Segment
- Thrusters are used when the CMGs can no longer provide the required energy for repositioning.
- Star Trackers are an optical device that is used to determine the orientation and altitude of the ISS with respect to the stars.
- GPS Receivers provide information about the location of the ISS.
- Rate Gyros provide information about the change in orientation of the ISS.
With the proper guidance and navigation system in place, the ISS needs to find a way to stay in orbit.
As the station orbits Earth at an altitude ranging from 370 to 460 kilometers, it is constantly slowed by atmospheric drag and must be re-boosted periodically to maintain its altitude. It must also be maneuvered to assist in the rendezvous and docking of visiting vehicles and avoid debris.
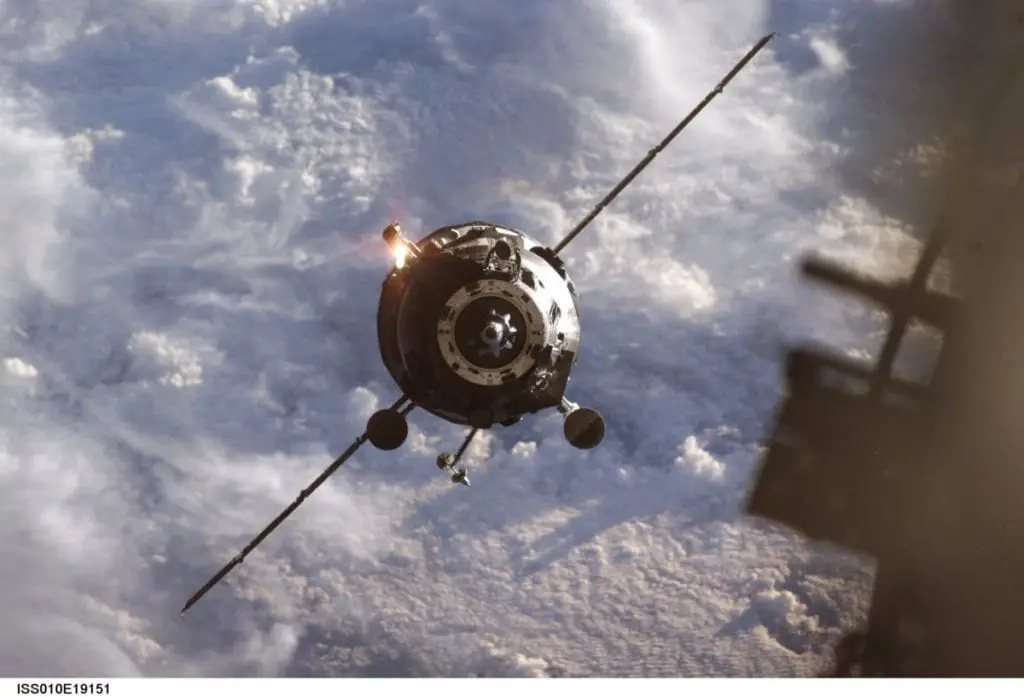
Image by NASA
Three rocket engines are used on the ISS for this purpose:
- Progress Rocket Engines
- Service Module Rocket Engines
- FGB Rocket Engines
How does the ISS avoid space debris?
Unfortunately, the International Space Station can’t avoid all space debris given its massive body. And there are millions of pieces of space junk flying in Low Earth Orbit.
Just in 2020, the ISS had maneuvered 3 times to avoid debris, with the latest to occur on September 22, 2020, when the ISS used thrusters of a cargo ship that was docked to the station, along with NASA and Russian flight controllers working together to avoid colliding with an unknown piece of debris.
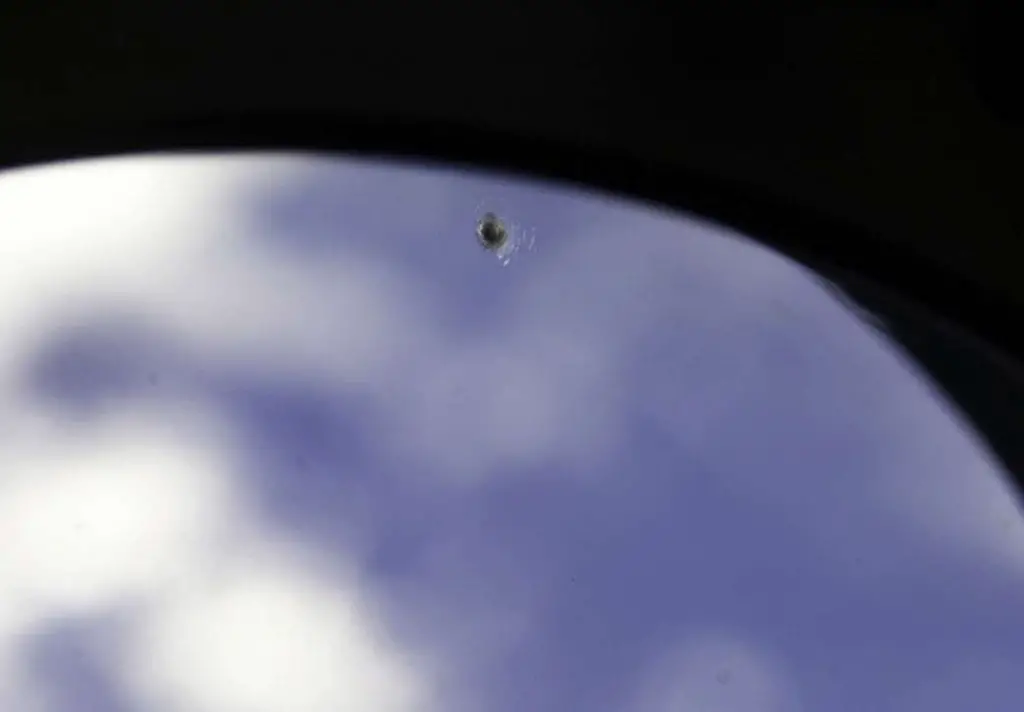
Image by NASA
Aside from active avoidance, the ISS has installed Micrometeoroid and Orbital Debris (MMOD) Protection to guard against debris that is too small or too fast to avoid.
The debris can approach the ISS in various threat directions, but it is less likely to come from below, with Earth acting as a shield. Most debris travels parallel with Earth’s surface and approaches the station from the side or front.
The following are the primary shields installed on the ISS as a part of MMOD Protection:
- Whipple Shield
- two-layered with an outer bumper – outer bumper intended to break up, melt, or vaporize a particle on impact
- usually made of aluminum
- spaced to put distance between the impact and the module pressure shell wall
- used where only a few MMOD impacts are expected – aft, nadir, and zenith areas of ISS
- Stuffed Whipple Shield
- consists of the basic Whipple Shield with an underlying blanket of Nextel Ceramic Cloth and Kevlar fabric
- further increases the distance between the impactor and the module pressure shell
- used where more MMOD impacts are expected – front, starboard, and port sides of ISS
- Multi-paned Windows
- separate and redundant pressure panes
- outer debris pane/shutter
References
- Communication Systems in the International Space Station (ISS). (2004). 55th International Astronautical Congress of the International Astronautical Federation, the International Academy of Astronautics, and the International Institute of Space Law, 1–2. https://doi.org/10.2514/6.iac-04-t.2.09
- NASA. (2015). Reference Guide to the International Space Station. https://www.nasa.gov/sites/default/files/atoms/files/np-2015-05-022-jsc-iss-guide-2015-update-111015-508c.pdf