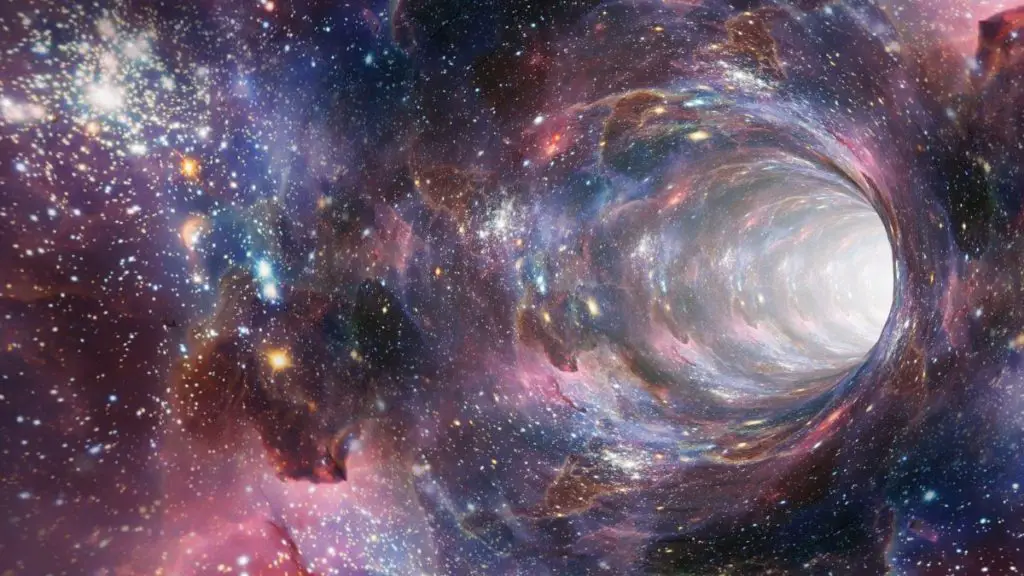
Understandably, the hope for humanity to reach another star within the next 100 years seem pretty slim. Whether it be for our lack of advanced propulsion systems or knowledge of interstellar travel, it might be better to just spend the rest of our lives traveling the planet God has created for us instead of looking out at the stars.
But what if I told you there was a way to cheat the system, and it will only take us seconds to reach the ends of the universe?
That’s right. Let’s talk wormholes.
In short, wormholes are the result of the spacetime continuum warping into a certain configuration that allows the linkage between two different points in spacetime. This could be to different locations, different points in time, or both.
The existence of wormholes would effectively allow us to travel any distance and to any point in time, as long as we learn how to control it. But how does it actually work?
What is a wormhole?
To understand what a wormhole is, we have to first adopt the view of spacetime existing as a topological structure instead of a flat space.
If you don’t quite have a grasp on the topic of spacetime topology – it is simply a structure that allows for the deformation of subspaces. An example would be how the gravitational field generated by a massive body can subjugate flat spacetime to a deformation, which results in its contraction and inward warp as a four-dimensional Lorentzian manifold.
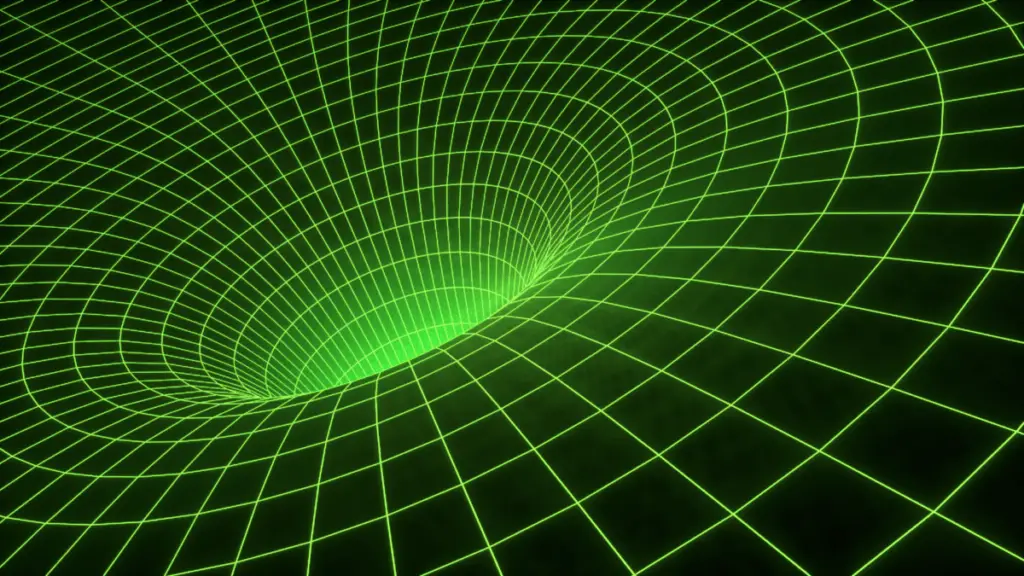
As such, a three-dimensional wormhole can be described as the topological connection between two separate regions of spacetime, where the “tube” or “bridge” that forms the connection exists as a subspace by deformation.
Einstein-Rosen Bridge
The theory for wormholes first came about in 1916 when an Austrian physicist by the name of Ludwig Flamm realized another solution was possible for Einstein’s theory of general relativity. He described the theoretical existence of a white hole (more on this below) with an entrance that was possibly connected to a black hole by a space-time conduit (Space).
Then in 1935, Einstein and Nathan Rosen elaborated on this idea to propose the existence of a “bridge” across space-time. This would in essence create a shortcut that would reduce travel time and distance across the universe. And this shortcut later came to be known as an Einstein-Rosen bridge (named after the two physicists). Or as we now know it – wormholes.
Are wormholes real?
Theoretically, the existence of wormholes is consistent with Einstein’s general theory of relativity, which says the observed gravitational effects between two masses are a result of their warping of spacetime. However, no one has ever seen one.
Well, at least not yet.
How can we find a wormhole?
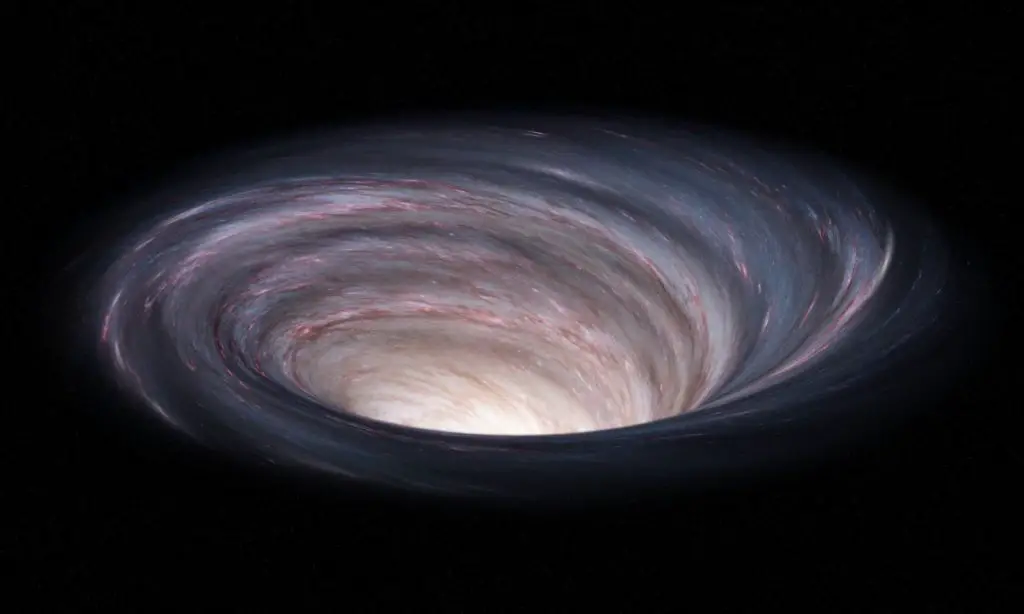
By definition, a wormhole smoothly connects two different regions of spacetime. But what could we expect to measure or look for in terms of astrophysical observations for such a portal?
Let’s use a traversable wormhole as an example.
First, flux properties.
The flux (scalar, electromagnetic, or gravitational) that pass through this wormhole can only be conserved in the totality of the two separate but connected regions of spacetime, not individually to their own (1). And the conservation of these flux properties will always adhere to the principle that in an isolated system (the universe), their net quantity never changes, or in other words – is always conserved. And this is assuming that the wormhole connected two points within the same universe of course.
Based on this understanding, suppose there is a physical electric charge on one side of the wormhole, and the observer of the other side sees the electric flux coming out – He would fairly conclude that the wormhole is just a charged object, and any measurements performed would not tell him otherwise (1). From the observer’s perspective, the electric flux might appear to be conserved in his space only, but we know that the flux has only traveled from one space to another.
If wormholes actually obey the laws of conservation, flux properties are probably a confusing metric to use as a standard in search of a wormhole.
What else can we do?
Well, let’s talk gravity.
It is actually possible to make astrophysical observations for a wormhole if there were time-dependent gravitational sources on both sides (i.e., orbiting star) (1). The observers of either side will no longer attribute the gravitational anomalies experienced as just another property of the wormhole, but actually consider the possibility for the existence of a “tunnel” that is connecting them to another region of spacetime.
As a result, the observers could begin the search for small deviations in the expected orbits of these orbiting stars, as you might think the objects within the wormhole’s vicinity on either side must be affected by each other’s gravitational field.
Since natural wormholes are expected to exist only in the most extreme conditions (e.g., around black holes), it would be the most promising for us to look for them where there are large black holes in the center of galaxies, or within a binary (double) black hole systems (1).
Currently, researchers are studying the motion of the star S2 which orbits the supermassive black hole Sagittarius A* at the center of our Milky Way Galaxy. They hope to “demonstrate that their near future data will be able to tell us if this black hole harbors a wormhole” (1).
Gravitational Lensing
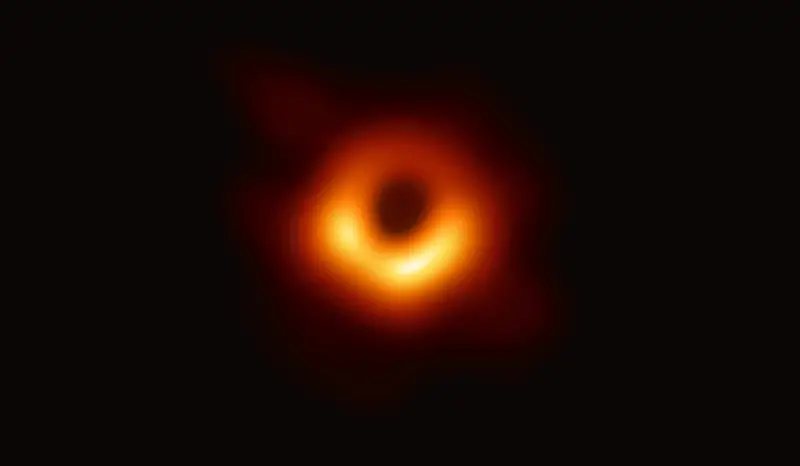
The gravitational lensing method is an effect used to find dark gravitating objects like black holes and wormholes.
When light emitted by stars far away from us pass by massive objects (i.e., black hole), its gravitational pull can deflect or bend the light. If the light source and the massive lensing object lie in a straight line with observers on Earth, the light source will appear as a ring around the massive object, given that this object has circular symmetry.
Other metrics we are able to rely on for observations are the gravitational lensing of light rays scattered by the throat of the wormhole or the photon sphere – the region where the gravitational pull is so strong that even light (photons) is forced to travel in an orbit (3).
But here lies the problem – we cannot effectively “distinguish wormholes with a positive mass from other massive objects such as a black hole” (3).
Unfortunately, I guess we are back to looking at orbits.
Wormhole vs. Black Hole
The basic difference between a wormhole and a black hole is that a black hole cannot be used for interstellar travel, while a wormhole can.
What is a black hole?
Unlike what we see on TV, black holes cannot be used for interstellar travel, and that is final. They are a one-way destination to a vacation spot called painful death.
Black holes have a horizon that separates their interior (where nothing can escape) and exterior (the universe we live in). This is known as a one-way membrane, where things can fall in, but nothing would come out.
So, two-way travel is by all sense strictly forbidden, and even considerations for one-way travel would require the object at the other end to be something a little more exotic, like a white hole.
Generally speaking, humans wouldn’t even be able to approach a black hole, much less go into one. The gravitational forces at the horizon are so enormous that a traveler approaching it would experience rapid acceleration at both their head and feet, which would eventually pull them apart into more pieces than we can count.
The only way (that we currently know of) to counteract this effect is to find a black hole that is 10,000 times more massive than the sun, with a horizon circumference of greater than 100,000 km. This would in turn distribute the gravity more proportionally across a human body to allow for safe passage into the interior of the black hole.
How are wormholes formed?
Dai D. C., Minic D. & Stojkovic D. (2020) published a paper in the European Physical Journal C that aimed to describe a possible mechanism of wormhole creation under the influence of classical instead of quantum gravity. This paper was written as there has been no realistic physical model of wormhole formation in the scientific community.
I will do my best to simply the presented concepts and give everyone an easy-to-understand step by step guide on how wormholes could be formed (under classical gravity).
In scientific literature, our universe has often been presented as a model of a 3+1-dimensional sub-space (the brane) that is embedded in a higher-dimensional space (the bulk). This is often known as the braneworld theory, which aims to explain the notion of how we are not able to perceive the extra dimensions beyond the four we are familiar with; these extra dimensions (the brane) that include our four-dimensional world actually exist within a higher-dimensional space (the bulk).
Because the branes could preserve their quantum fluctuations (temporary random changes in the amount of energy at a point in space), this uncertainty allows the branes to fold, twist and even cross themselves.
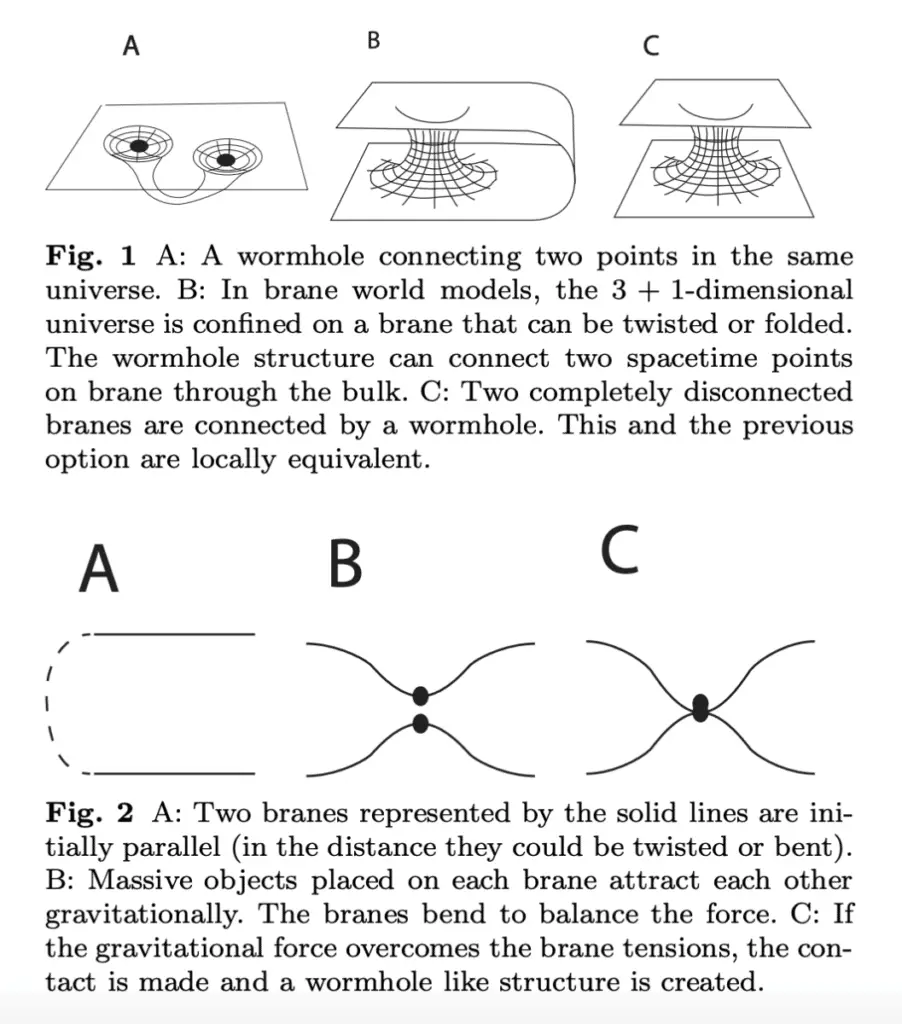
Three models were presented in this paper on the different ways a wormhole structure can connect between two points in spacetime (see Figure 1).
If we place two massive objects in separate parallel universes (modeled by two different branes), with a sufficiently strong gravitational attraction, these objects are able to break the resistance from the brane tension and cause a deformation that allows the objects to “touch”, thereby forming a wormhole (see Figure 2).
These conditions are more likely to be fulfilled the more massive and compact the objects are. So, we should be looking for wormholes “either in the background of black holes and compact stars, or massive-microscopic relics (microscopic blackholes)”, as they cause the highest amount of warp and deformation to spacetime.
Creating a Traversable Wormhole
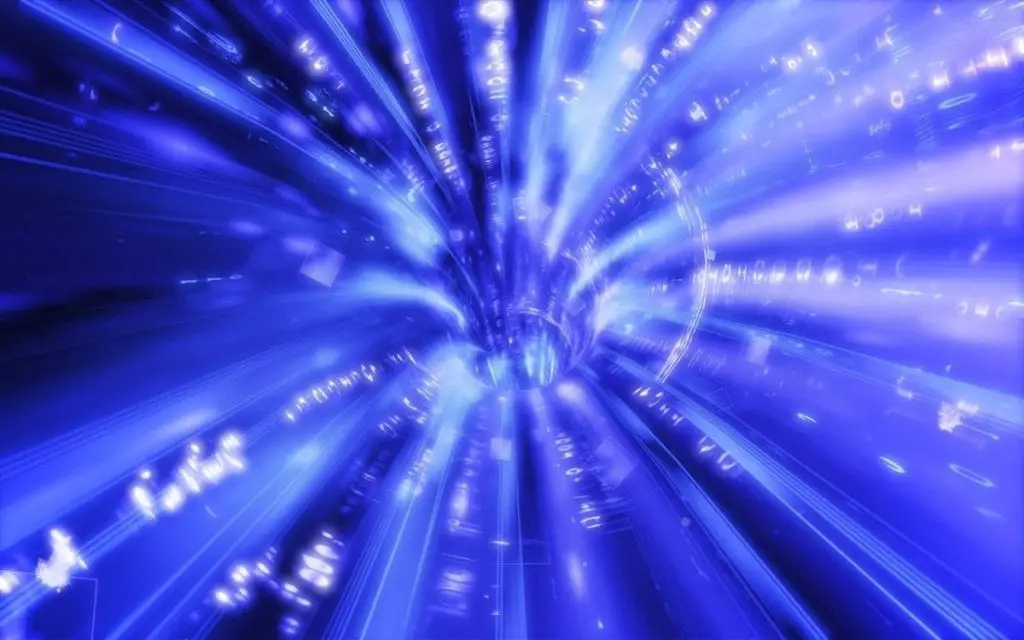
Several methods have been proposed to stabilize a wormhole that allows for travel, and the general consensus is that negative energy is required.
Negative Mass
Wormholes as a theoretical phenomenon are characteristically unstable and have a tendency to pinch off and disappear.
Wormholes generally have a delicate balance between positive and negative energy; adding a small amount of either will tip the scale and cause it to collapse. If you were to enter a wormhole, this contribution of positive mass/energy would destabilize and distort the “tunnel”, and the entrance would snap itself shut, trapping (probably also kill) you in a subspace located at who knows where.
But if we think about this logically, as long as we keep the balance of energy, everything will be okay?
Theoretically yes, that’s exactly how it would work. However, this would require us to throw in some negative mass along with whoever decided to take the trip.
Negative mass is a hypothetical concept of matter whose mass is pretty much just of opposite sign to the mass of normal matter, and we have unfortunately not discovered any.
Negative mass in theory would generate repulsive gravitational sources and repel positive mass (ordinary matter), as well as attract each other to form negative massive clumps (4). If we took the time to look, we might be able to find these clumps in the cosmological voids of the universe.
White Holes
Just by its name, white holes sound awfully similar to what we know as black holes… If that’s the connection you made, then you are completely right!
A white hole is a theoretical existence with properties completely opposite to that of a black hole. Whereas a black hole won’t let anything out, a white hole will not let anything in.
With a white hole acting as a compact body of some mass M – its mass will decrease as matter continuously pours out with nothing capable of entering to replenish it. It is from such a model that scientists have concluded that white holes are extremely unstable and will inevitably convert themselves into a black hole (5).
With regards to the formation of a wormhole, we need two massive objects that have the ability to distort spacetime to an effect where a subspace tunnel is formed between them. Since we need an entrance and an exit, a black and white hole seems the perfect candidate. However, it is important to know this would only allow the formation of a one-way traversable wormhole, not a two-way.
And unfortunately, just like negative mass, we have not found any evidence for the existence of white holes, but they are a possibility.
Charged Black Holes and Cosmic Strings
Let’s talk charged black holes.
Generally speaking, natural forming black holes do not possess electric charge due to the amount of electromagnetic repulsion required to compress an electrically charged mass, which is greater than the natural gravitational attraction of the black hole. So, we cannot actually find them in the universe, but they do exist within the realm of theoretical physics and mathematics.
There are two types of charged black holes, both named after the physicist that solved them:
- Reissner-Nordström – no angular momentum but has electric charge
- Kerr-Newman – has both angular momentum and electric charge
In a paper published by Fu. Z., Grado-White. B. & Marolf. D. (2019), they proposed the theoretical construction of a traversable wormhole with a pair of oppositely charged blackholes (Reissner-Nordström), which at first will be connected by a non-traversable wormhole.
To prevent the two black holes from merging due to their gravitational AND newly added electric forces, as it would just result in a bigger but neutrally charged black hole – we have to make sure they stay sufficiently far enough from each other to keep the wormhole open.
As it stands right now, we need two solutions. One to keep the two black holes away from each other, and another to make sure the wormhole is traversable.
The solution is simple really – we will use not one, but two cosmic strings.
I know what you’re thinking, they didn’t teach me about cosmic strings in high school either. It literally sounds like it came straight out of a Star Wars movie. But, after a little bit of research, I think I have an answer.
Cosmic strings are hypothetical one-dimensional defects formed during the phase transitions of the early universe.
When the universe was first created, it began in a unified or ‘symmetric’ phase that gradually got hotter and denser. This increase in energy resulted in a succession of phase transitions, which until finally, at low enough temperatures, the universe was stabilized as matter particles like the ones we are familiar with today: electrons, protons, neutrons, etc.
During these phase changes, just like how cracks might form when water turns into ice, so did the cosmic strings.
The first string will be threaded through the wormhole and stretched to infinity as the string’s tension prevents the charged black holes from being attracted to each other. The second will also be threaded but this time closed in a loop. When a cosmic string is closed in a loop, its quantum fluctuations (vibrations) provide the negative energy/mass required to stabilize the wormhole for travel.
Unfortunately, this still wouldn’t be able to keep the wormhole open for as long as we would like. The mass of the cosmic string will continuously deplete as more and more energy is pulled away to stabilize the wormhole. And as all good things come to an end, so will the cosmic strings.
References
- Dai, D.-C., & Stojkovic, D. (2019). Observing a wormhole. Physical Review D, 100(8), 1–7. https://doi.org/10.1103/physrevd.100.083513
- Dai, D.-C., Minic, D., & Stojkovic, D. (2020). How to form a wormhole. The European Physical Journal C, 80(12), 1–6. https://doi.org/10.1140/epjc/s10052-020-08698-x
- Tsukamoto, N. (2020). Nonlogarithmic divergence of a deflection angle by a marginally unstable photon sphere of the Damour-Solodukhin wormhole in a strong deflection limit. Physical Review D, 101(10), 1–13. https://doi.org/10.1103/physrevd.101.104021
- Takahashi, R., & Asada, H. (2013). OBSERVATIONAL UPPER BOUND ON THE COSMIC ABUNDANCES OF NEGATIVE-MASS COMPACT OBJECTS AND ELLIS WORMHOLES FROM THE SLOAN DIGITAL SKY SURVEY QUASAR LENS SEARCH. The Astrophysical Journal, 768(1), L16. https://doi.org/10.1088/2041-8205/768/1/l16
- Eardley, D. M. (1974). Death of White Holes in the Early Universe. Physical Review Letters, 33(7), 442–444. https://doi.org/10.1103/physrevlett.33.442
- Fu, Z., Grado-White, B., & Marolf, D. (2019). Traversable asymptotically flat wormholes with short transit times. Classical and Quantum Gravity, 36(24), 245018. https://doi.org/10.1088/1361-6382/ab56e4
- Morris, M. S., & Thorne, K. S. (1988). Wormholes in spacetime and their use for interstellar travel: A tool for teaching general relativity. American Journal of Physics, 56(5), 395–412. https://doi.org/10.1119/1.15620